Part Two: How’s Your Latin?
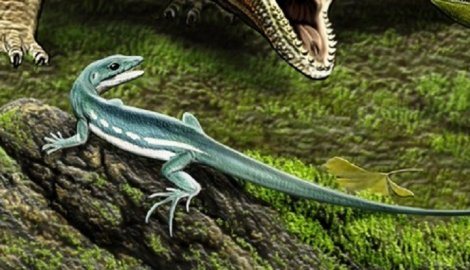
What do Barack Obama, Marco Polo, and the band Green Day have in common? They all have at least one organism named after them. Obama has several, including a bird called Nystalus obamai and an extinct reptile named Obamadon gracilis. Green Day’s honorary organism is the plant Macrocarpaea dies-viridis, “dies-viridis” being Latin for “green day.” Many scientists also have species named after them, usually as recognition for their contributions to a field. My own PhD advisor, Dr. Anne Bruneau, has a genus of legumes, Annea, named after her for her work in legume systematics.
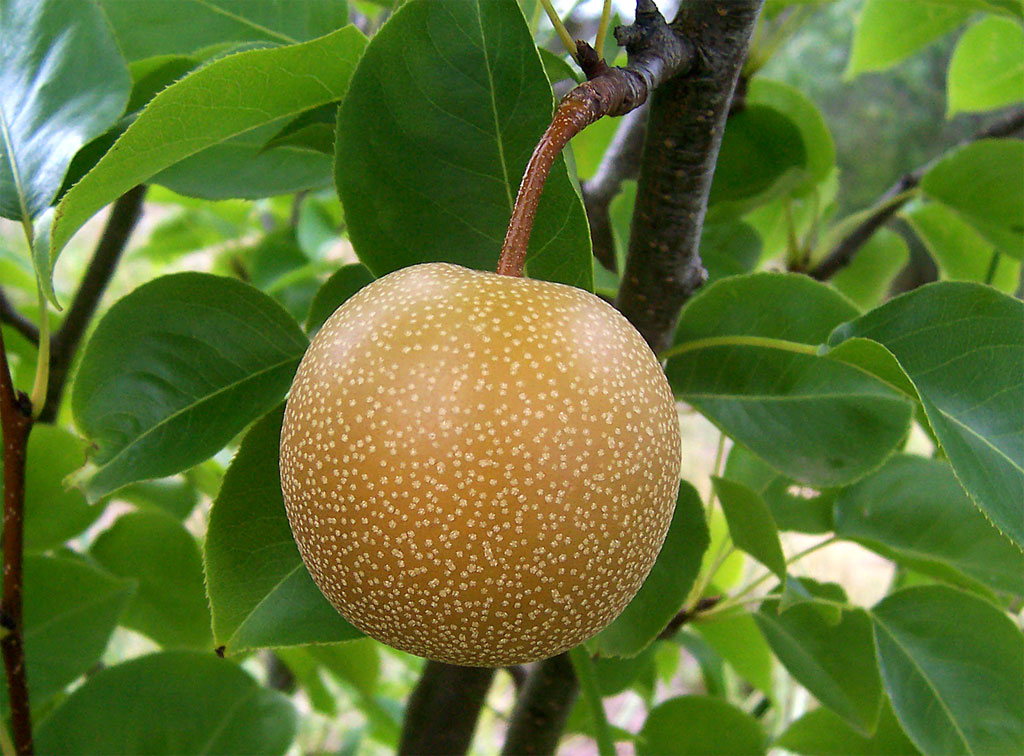
Scientific names, which are colloquially called Latin names, but which often draw from Greek as well, consist of two parts: the genus, and the specific epithet. The two parts together are called the species. Though many well-known scientists, celebrities, and other note-worthies do have species named after them, most specific epithets are descriptive of some element of the organism or its life cycle. Many of these are useful descriptions, such as the (not so bald) bald eagle, whose scientific name is the more accurate Haliaeetus leucocephalus, which translates to “white-headed sea eagle.” (See here for some more interesting examples.) A few are just botanists being hilariously lazy with names, as in the case of Pyrus pyrifolia, the Asian pear, whose name translates as “pear-leaved pear.” So we know that this pear tree has leaves like those of pear trees. Great.
In contrast to common names, discussed in our last post, Latin names are much less changeable over time, and do not have local variants. Soybeans are known to scientists as Glycine max all over the world, and this provides a common understanding for researchers who do not speak the same language. Latin is a good base language for scientific description because it’s a dead language, and so its usage and meanings don’t shift over time the way living languages do. Until recently, all new plant species had to be officially described in Latin in order to be recognized. Increasingly now, though, descriptions in only English are being accepted. Whether this is a good idea remains to be seen, since English usage may shift enough over the years to make today’s descriptions inaccurate in a few centuries’ time.
This isn’t to say that scientific names don’t change at all. Because scientific names are based in organisms’ evolutionary relationships to one another (with very closely related species sharing a genus, for example), if our understanding of those relationships changes, the name must change, too. Sometimes, this causes controversy. The most contentious such case in the botanical world has been the recent splitting of the genus Acacia.
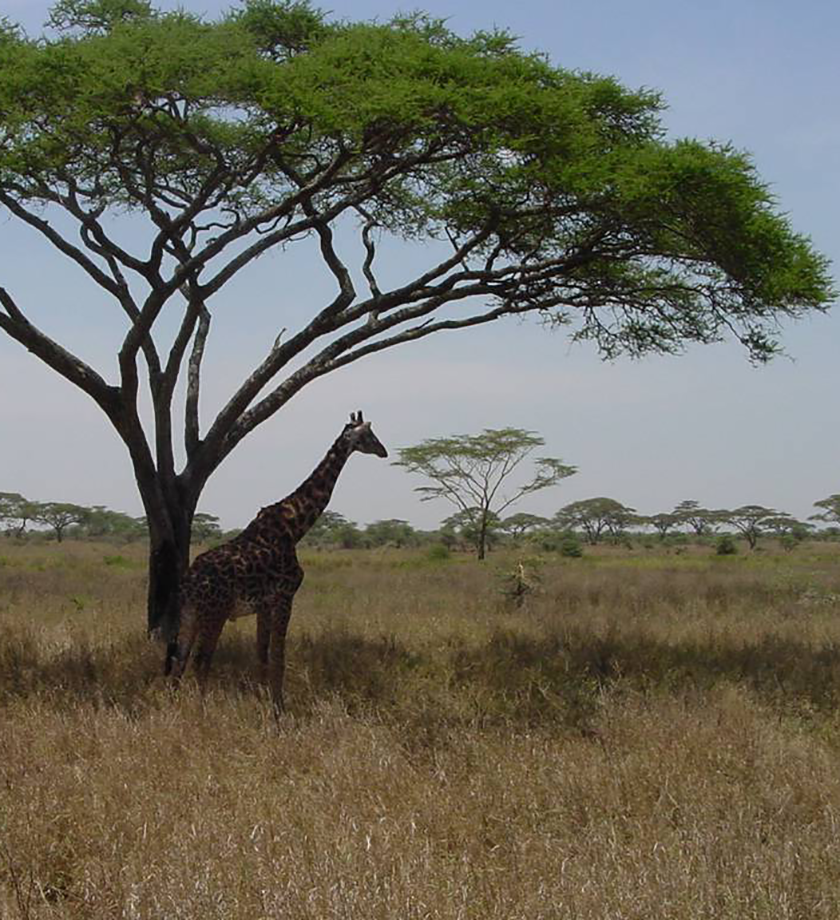
Acacia is/was a large genus of legumes found primarily in Africa and Australia (discussed previously on this blog for their cool symbiosis with ants). In Africa, where the genus was first created and described, the tree is iconic. The image of the short, flat-topped tree against a savanna sunset, perhaps accompanied by the silhouette of a giraffe or elephant, is a visual shorthand for southern Africa in the popular imagination, and has been used in many tourism campaigns. The vast majority of species in the genus, however, are found in Australia, where they are known as wattles. When it became apparent that these sub-groups needed to be split into two different genera, one or the other was going to have to give up the name. A motion was put forth at the International Botanical Congress (IBC) in Vienna in 2005 to have the Australian species retain the name Acacia, because fewer total species would have to be renamed that way. Many African botanists and those with a stake in the acacias of Africa objected. After all, African acacias were the original acacias. The motion was passed, however, then challenged and upheld again at the next IBC in Melbourne in 2011. (As a PhD student in legume biology at the time, I recall people having firm and passionate opinions on this subject, which was a regular topic of debate at conferences.) It is possible it will come up again at this year’s IBC in China. Failing a major turnaround, though, the 80 or so African acacias are now known as Vachellia, while the over one thousand species of Australian acacias continue to be known as Acacia.
The point of this story is, though Latin names may seem unchanging and of little importance other than a means of cataloguing species, they are sometimes both a topic of lively debate and an adaptable reflection of our scientific understanding of the world.
Do you have a favourite weird or interesting Latin species name? Make a comment and let me know!